8.06 Haemoglobin (Bohr + Diss. Curve)

Haemoglobin Structure and Function
- Composition:
- Haemoglobin is a protein composed of four polypeptide chains, each containing one haem group that can bind one O₂ molecule.
- Oxygen Binding:
- Each haemoglobin molecule can bind up to four O₂ molecules (Hb + 4O₂ → HbO₄).
- Role:
- Transports oxygen from the lungs to body tissues for aerobic respiration.

Key Terms
- Partial Pressure:
- A measure of the concentration of a gas in a mixture of gases.
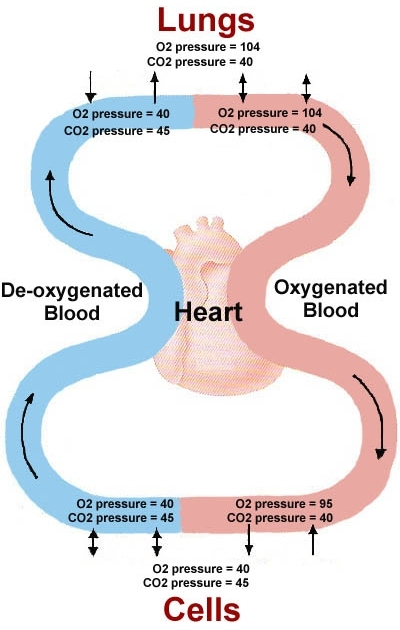
- Percentage Saturation:
- The percentage of haemoglobin molecules that are bound with oxygen.
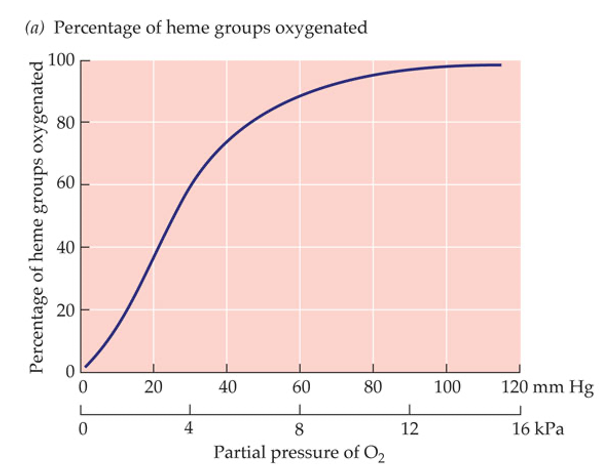
- Dissociation Curve:
- A graph showing haemoglobin’s oxygen saturation versus oxygen partial pressure.
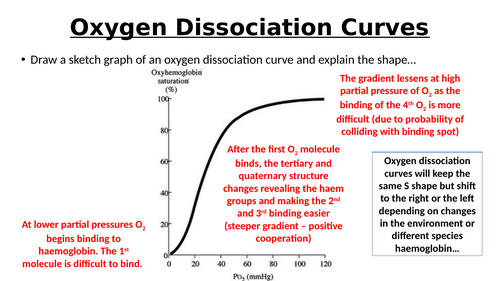
Haemoglobin Dissociation Curve
- Characteristics:
- S-shaped Curve:
- Low O₂ pressures: Haemoglobin has low saturation.
- High O₂ pressures: Haemoglobin approaches full saturation (95-97% in lungs).
- S-shaped Curve:
- Behavior:
- In Lungs (High O₂ Pressure):
- Haemoglobin binds O₂ effectively.
- In Tissues (Low O₂ Pressure):
- Haemoglobin releases O₂ (e.g., muscles at ~20-25% saturation).
- In Lungs (High O₂ Pressure):
- Cooperative Binding:
- Binding of one O₂ molecule changes haemoglobin’s shape, making it easier for additional O₂ molecules to bind, resulting in the steep rise in the curve.
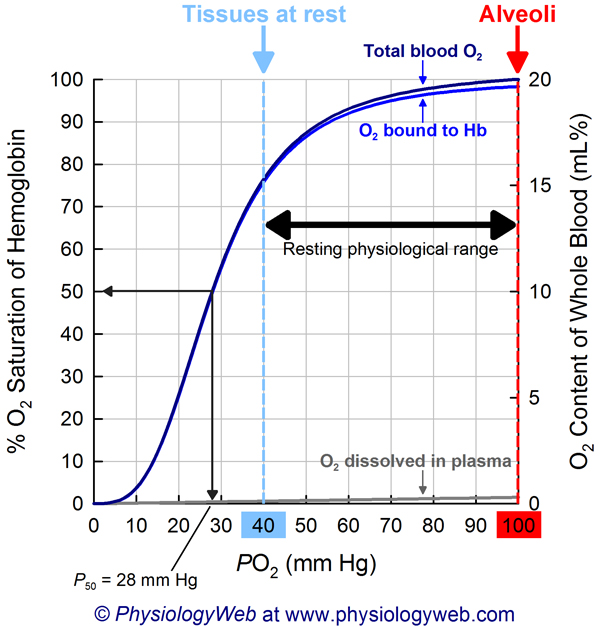

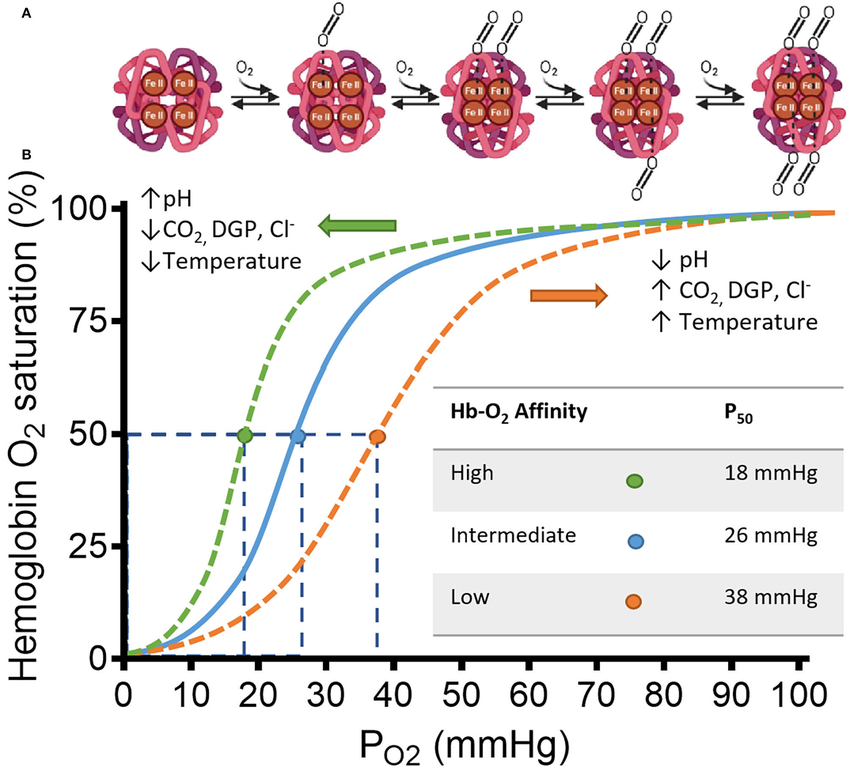
Example: Step by step
. 1.Oxygen Loading in the Lungs
- High pO₂ Environment: The alveoli in the lungs have a high partial pressure of oxygen (approximately 100 mmHg).
- Hemoglobin Binding: Under these conditions, hemoglobin molecules in red blood cells bind oxygen efficiently. This is represented by the flat, upper portion of the dissociation curve where hemoglobin saturation is high (around 97-100%).
- Oxygen Saturation: Most hemoglobin molecules are saturated with oxygen, resulting in oxygen-rich blood being pumped from the lungs into the systemic circulation.
2. Transporting Oxygen to Tissues
- Systemic Circulation: Oxygen-rich blood travels through the arteries to various tissues in the body.
- Stable pO₂ Gradient: As blood moves away from the lungs, it encounters tissues with lower pO₂ compared to the alveoli, creating a gradient that facilitates oxygen release.
3. Oxygen Release in Resting Tissues
- Moderate pO₂ Environment: Resting tissues have a pO₂ of about 40 mmHg.
- Hemoglobin Saturation: At this pO₂, hemoglobin releases some oxygen, reducing its saturation to approximately 75% (mid-portion of the curve).
- Adequate Oxygen Supply: The amount of oxygen released is sufficient to meet the metabolic demands of tissues at rest.
- Returning Blood: After oxygen delivery, blood returns to the lungs with a relatively high oxygen content because not all oxygen was released in resting tissues.
4. Oxygen Release in Exercising Tissues
- Lower pO₂ Environment: Exercising muscles generate more carbon dioxide and produce more metabolic byproducts, leading to a drop in pO₂ (down to about 20 mmHg).
- Shift in the Curve (Bohr Effect): Increased carbon dioxide and lower pH cause the dissociation curve to shift to the right, facilitating greater oxygen release.
- Hemoglobin Saturation: At lower pO₂, hemoglobin releases more oxygen, reducing its saturation further (possibly down to 50-60%).
- Enhanced Oxygen Delivery: This ensures that actively exercising tissues receive more oxygen to meet higher metabolic demands.
- Returning Blood: Blood returning from exercising tissues has lower oxygen content compared to blood from resting tissues because more oxygen was released to meet the increased needs.
5. Implications for Oxygen Delivery and Return Blood Oxygen Levels
- During Exercise: The increased demand for oxygen in exercising tissues leads to greater oxygen release, resulting in blood returning with significantly lower oxygen content.
- At Rest: Since resting tissues have a moderate demand for oxygen, hemoglobin releases enough oxygen to meet these needs without a drastic drop in saturation. Consequently, blood returning to the lungs from resting tissues retains a higher oxygen content.
The Bohr Shift
Introduction
- The Bohr Shift is a critical physiological mechanism that facilitates efficient oxygen delivery to tissues based on their metabolic activity.
- Named after the Danish physiologist Christian Bohr, who first described the phenomenon in 1904, the Bohr Shift explains how hemoglobin’s affinity for oxygen changes in response to varying concentrations of carbon dioxide (CO₂) and pH levels within the blood.
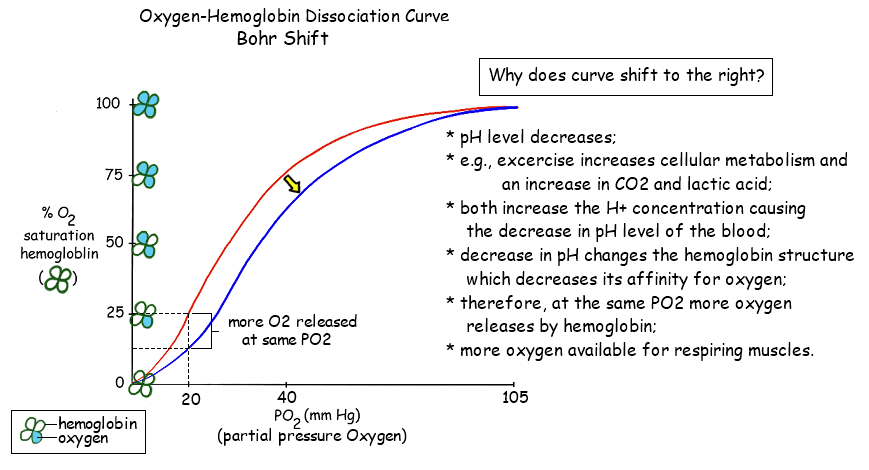
Definition
- Bohr Shift:
A shift in the oxygen-hemoglobin dissociation curve caused by changes in blood pH and CO₂ concentration, resulting in decreased hemoglobin affinity for oxygen and enhanced oxygen release to tissues.
Hemoglobin and Oxygen Binding
- Hemoglobin (Hb):
A protein in red blood cells responsible for transporting oxygen from the lungs to body tissues and facilitating the return transport of carbon dioxide from tissues to the lungs. - Oxygen-Hemoglobin Dissociation Curve:
A graph that depicts the relationship between the partial pressure of oxygen (pO₂) and the percentage saturation of hemoglobin with oxygen. The curve is sigmoidal (S-shaped), indicating cooperative binding.
Mechanism of the Bohr Shift
Factors Influencing the Bohr Shift
- Carbon Dioxide (CO₂) Concentration:
- Increased CO₂:
- Produced by active tissues during metabolism.
- Diffuses into red blood cells and reacts with water to form carbonic acid (H₂CO₃) via the enzyme carbonic anhydrase.
- H₂CO₃ dissociates into bicarbonate ions (HCO₃⁻) and hydrogen ions (H⁺).
- Increased CO₂:
- pH Levels:
- Decreased pH (Acidosis):
- Higher concentration of H⁺ ions lowers blood pH.
- H⁺ ions bind to hemoglobin, causing conformational changes that reduce hemoglobin’s affinity for oxygen.
- Decreased pH (Acidosis):
- Temperature:
- Increased Temperature:
- Often accompanies active metabolism.
- Enhances the Bohr Shift by further decreasing hemoglobin’s oxygen affinity.
- Increased Temperature:
Detailed Process
- At Active Tissues:
- High Metabolic Activity:
- Produces more CO₂ and heat.
- Leads to increased CO₂ concentration and decreased pH in the surrounding blood.
- High Metabolic Activity:
- Within Red Blood Cells:
- CO₂ Conversion:
- CO₂ + H₂O ↔ H₂CO₃ (catalyzed by carbonic anhydrase) ↔ H⁺ + HCO₃⁻.
- CO₂ Conversion:
- Effect on Hemoglobin:
- H⁺ Binding:
- H⁺ ions attach to hemoglobin, stabilizing the T-state (low oxygen affinity).
- Conformational Change:
- Haemoglobin’s structure goes through a change, which shifts it from being a molecule with a high oxygen affinity to one with a lower affinity, facilitating oxygen release.
- H⁺ Binding:
- Oxygen Release:
- Enhanced Dissociation:
- Reduced affinity allows hemoglobin to release more oxygen where it’s needed most.
- Enhanced Dissociation:
Physiological Importance
- Efficient Oxygen Delivery:
Ensures that oxygen is preferentially released in tissues with high metabolic demands, such as muscles during exercise or active brain regions. - Adaptation to Metabolic Changes:
Allows the circulatory system to dynamically adjust oxygen delivery based on real-time metabolic activity and environmental conditions. - Coordination with Respiratory System:
Promotes the uptake of CO₂ in the tissues and its removal via the lungs, maintaining acid-base balance in the blood.
The Oxygen-Hemoglobin Dissociation Curve and the Bohr Shift
- Shifts in the Curve:
- Right Shift (Bohr Shift):
- Caused by increased CO₂, decreased pH, increased temperature.
- Indicates decreased affinity of hemoglobin for oxygen, promoting oxygen release.
- Left Shift:
- Caused by decreased CO₂, increased pH, decreased temperature.
- Indicates increased affinity of hemoglobin for oxygen, promoting oxygen uptake.
- Right Shift (Bohr Shift):
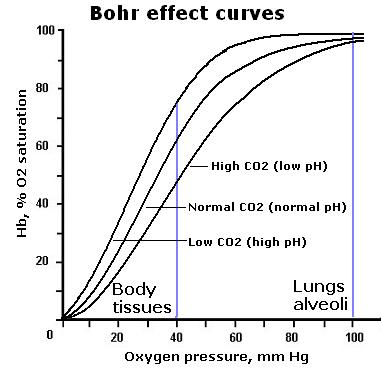
Mathematical Representation
- Hill Equation:
Describes the cooperativity of oxygen binding to hemoglobin.

- Y: Fraction of oxygen-bound hemoglobin.
- pO₂: Partial pressure of oxygen.
- P₅₀: Partial pressure at which hemoglobin is 50% saturated.
- n: (the Hill coefficient) quantifies the degree of cooperativity in binding (for human hemoglobin). It is typically around 3).
- S is the fractional saturation of hemoglobin (i.e. the fraction of binding sites occupied by oxygen),
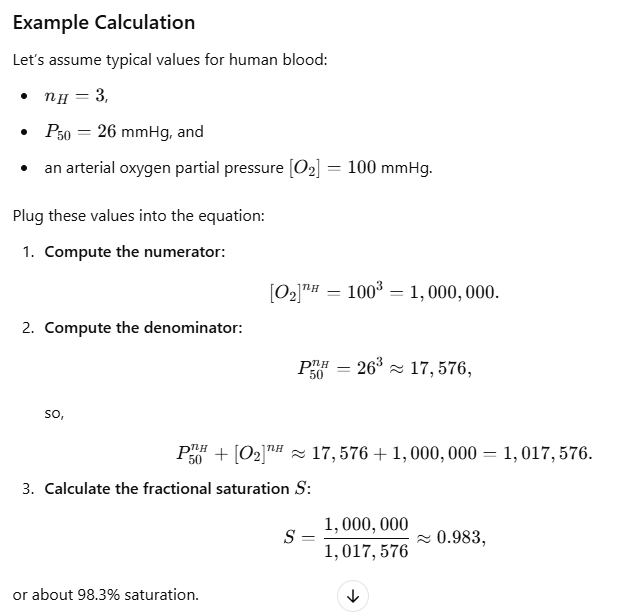
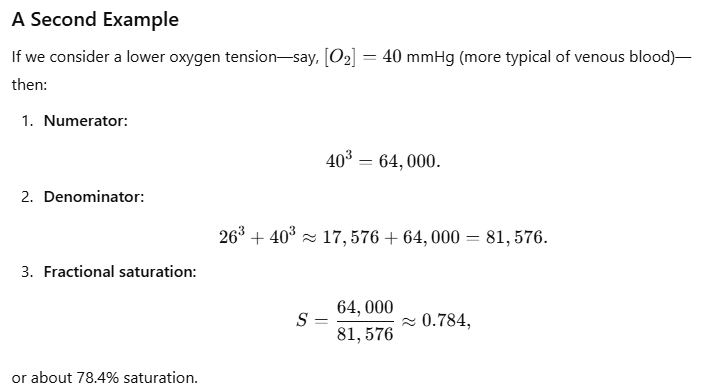
- Impact of Bohr Shift on P₅₀:
- Right Shift:
- Increases P₅₀, meaning higher pO₂ is required for 50% saturation, indicating lower affinity.
- Left Shift:
- Decreases P₅₀, meaning lower pO₂ is required for 50% saturation, indicating higher affinity.
- Right Shift:
Clinical Relevance
- Chronic Obstructive Pulmonary Disease (COPD):
- Impact:
- Impaired gas exchange leads to increased CO₂ levels.
- Enhances the Bohr Shift, promoting oxygen release to compensate for reduced oxygen uptake.
- Impact:
- Metabolic Acidosis:
- Definition:
- Condition characterized by decreased blood pH due to excessive acid production or loss.
- Effect:
- Amplifies the Bohr Shift, increasing oxygen delivery to tissues.
- Definition:
- Carbon Monoxide Poisoning:
- Mechanism:
- CO binds to hemoglobin with higher affinity than oxygen, reducing oxygen delivery.
- Relation to Bohr Shift:
- The presence of CO exacerbates the Bohr Shift effects by further decreasing hemoglobin’s oxygen affinity.
- Mechanism:
- Sickle Cell Anemia:
- Effect on Oxygen Release:
- Abnormal hemoglobin structure affects the Bohr Shift, impairing oxygen delivery to tissues.
- Effect on Oxygen Release:
Fetal Hemoglobin (HbF) and the Bohr Shift
- Fetal Hemoglobin (HbF):
- Higher Oxygen Affinity:
- Facilitates efficient oxygen uptake from maternal blood across the placenta.
- Higher Oxygen Affinity:
- Bohr Shift in Fetuses:
- Enhanced Oxygen Delivery:
- The Bohr Shift ensures that oxygen is preferentially released to fetal tissues, complementing the high affinity of HbF for oxygen.
- Enhanced Oxygen Delivery:
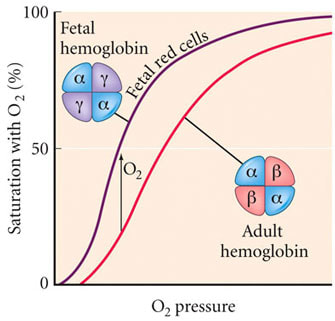
Mechanism of the Bohr Effect in Muscle Tissue
A. Conditions in Active Muscle Tissue
- High CO₂ Production: Muscle cells produce CO₂ as a by-product of aerobic metabolism.
- Increased H⁺ Concentration: CO₂ reacts with water to form carbonic acid (H₂CO₃), which dissociates into H⁺ and bicarbonate ions (HCO₃⁻), lowering pH.
- Lower pH (Acidosis): Accumulation of H⁺ ions decreases the pH in the local environment.
B. Impact on Hemoglobin (Hb)
- Reduced O₂ Affinity: Elevated CO₂ and H⁺ bind to hemoglobin, inducing conformational changes that decrease Hb’s affinity for O₂.
- Enhanced O₂ Release: With lower affinity, O₂ is more readily released from hemoglobin to meet the metabolic demands of muscle cells.
Physiological Significance
- Efficient Oxygen Delivery: Ensures that active muscles receive more O₂ when they need it most during increased activity.
- Facilitation of CO₂ Removal: Promotes the uptake of CO₂ from muscle cells for transport back to the lungs.
Key Factors Influencing the Bohr Effect in Muscles
- CO₂ Levels: Higher CO₂ concentration enhances the Bohr Effect.
- pH Levels: Lower pH (higher H⁺ concentration) strengthens the Bohr Effect.
- Temperature: Increased temperature in active muscles can further reduce Hb’s O₂ affinity.
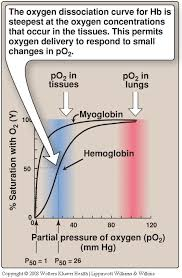
Oxygen Dissociation Curves: Myoglobin vs. Hemoglobin

- Myoglobin Curve:
- Hyperbolic shape.
- Myoglobin has a very high affinity for oxygen and binds it strongly, even at low partial pressures of oxygen (pO₂).
- This high affinity ensures myoglobin effectively picks up oxygen released by hemoglobin in tissues.
- Hemoglobin Curve:
- Sigmoidal shape due to cooperative binding (oxygen binding increases affinity for subsequent oxygen molecules).
- At the tissue level, hemoglobin’s oxygen affinity is reduced by factors such as:
- Higher carbon dioxide levels.
- Lower pH (Bohr effect).
- Higher temperature.
- Presence of 2,3-bisphosphoglycerate (2,3-BPG).
Why is Myoglobin’s Curve Left-Shifted?
- Higher Oxygen Affinity:
- Myoglobin’s role is to store oxygen in muscles and release it only when pO₂ is critically low (e.g., during intense exercise).
- This requires it to bind oxygen strongly, even when hemoglobin is releasing it.
- Non-Cooperative Binding:
- Myoglobin has a single heme group and does not exhibit cooperative binding, unlike hemoglobin. Its oxygen-binding affinity remains consistently high across a range of pO₂ values.
- Comparison to Tissue-Level Hemoglobin:
- In tissues, hemoglobin’s dissociation curve shifts rightward due to the Bohr effect (lower pH, higher CO₂), reducing its oxygen affinity.
- Myoglobin’s higher affinity ensures it can still extract oxygen released by hemoglobin under these conditions.
Physiological Significance
- The left-shifted curve of myoglobin relative to hemoglobin ensures:
- Efficient oxygen transfer from hemoglobin (lower affinity) to myoglobin (higher affinity) in the tissues.
- Oxygen is retained in muscle cells until critically needed during hypoxia or high-energy demand.
Bohr compared with the Haldane Effect
Haldane Effect:
- The Haldane Effect describes the influence of oxygenation on hemoglobin’s ability to bind and transport carbon dioxide and protons (H⁺).
- Specifically, it refers to the increased capacity of deoxygenated hemoglobin to carry CO₂ and H⁺, and conversely, the reduced ability of oxygenated hemoglobin to carry these molecules.
Key Points:
- Deoxygenated Hemoglobin (Hb): Binds more CO₂ and H⁺.
- Oxygenated Hemoglobin (HbO₂): Binds less CO₂ and H⁺.
- This effect facilitates the uptake of CO₂ in tissues and its release in the lungs, enhancing overall gas exchange efficiency.
2. Mechanism of the Haldane Effect

a. CO₂ Transport in the Blood
CO₂ is transported in the blood in three primary forms:
- Dissolved CO₂: Approximately 5-10% of total CO₂ is dissolved directly in plasma.
- Carbaminohemoglobin (HbCO₂): About 20-30% binds directly to hemoglobin.
- Bicarbonate Ions (HCO₃⁻): The majority (60-70%) is converted to bicarbonate ions in plasma.
b. Role of Hemoglobin in CO₂ Transport
- Deoxygenated Hemoglobin:
- Enhanced CO₂ Binding: When hemoglobin releases oxygen to the tissues, it becomes deoxygenated (Hb). This state increases its affinity for CO₂ and H⁺, promoting the formation of carbaminohemoglobin and facilitating the conversion of CO₂ to bicarbonate.
- Chloride Shift: As bicarbonate ions accumulate in red blood cells, they are exchanged for chloride ions (Cl⁻) in plasma to maintain electrical neutrality, a process known as the chloride shift.
- Oxygenated Hemoglobin:
- Reduced CO₂ Binding: In the lungs, oxygen binds to hemoglobin, converting it to oxyhemoglobin (HbO₂). This oxygenation decreases hemoglobin’s affinity for CO₂ and H⁺, promoting the release of CO₂ from hemoglobin.
- Facilitated CO₂ Release: Lower CO₂ affinity in the lungs aids in the expulsion of CO₂ during exhalation.
c. Interaction with Protons (H⁺)
- Deoxygenated Hemoglobin:
- Buffering Capacity: Deoxygenated hemoglobin can bind free H⁺ ions, helping to buffer blood pH by mitigating acidity.
- Oxygenated Hemoglobin:
- Reduced Buffering: Oxygenated hemoglobin has a decreased ability to bind H⁺, which can influence blood pH regulation.
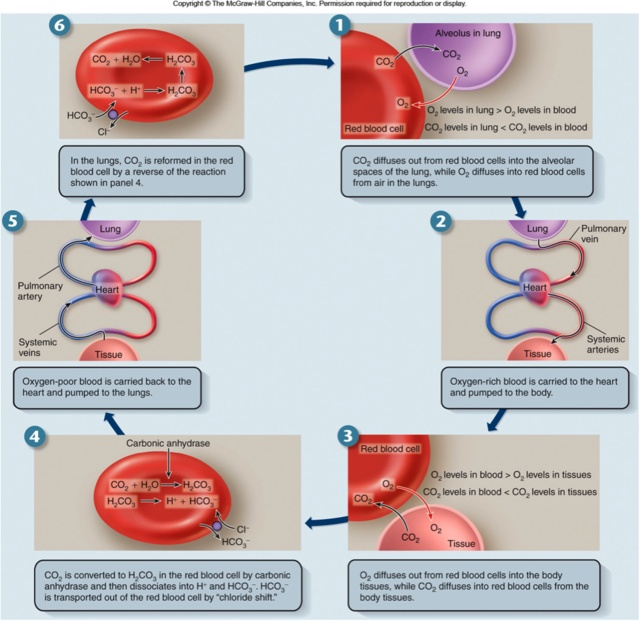
3. Physiological Significance of the Haldane Effect
a. Enhanced Gas Exchange Efficiency
- In Tissues (Peripheral):
- Oxygen Release: Oxygen is released from hemoglobin to meet metabolic demands.
- CO₂ Uptake: Simultaneously, the deoxygenated state of hemoglobin enhances CO₂ binding and transport from tissues to the blood.
- In Lungs (Pulmonary):
- Oxygen Uptake: Oxygen binds to hemoglobin, increasing its oxygen saturation.
- CO₂ Release: The oxygenated state reduces hemoglobin’s affinity for CO₂, facilitating its release into the alveoli for exhalation.
b. Maintenance of Blood pH
- Buffering Action: By binding H⁺ ions, deoxygenated hemoglobin helps maintain blood pH, especially in actively metabolizing tissues where lactic acid production can increase acidity.
c. Complementary Role with the Bohr Effect
- Bohr Effect: Describes how increased CO₂ and H⁺ concentrations (lower pH) in tissues decrease hemoglobin’s affinity for oxygen, promoting oxygen release.
- Haldane Effect: Complements the Bohr Effect by enhancing CO₂ uptake in deoxygenated blood and facilitating its release in oxygenated blood.
Together, these effects ensure that oxygen delivery and CO₂ removal are tightly coupled to the metabolic activity of tissues.
Fetal Hemoglobin (HbF)
Definition
- Fetal Hemoglobin (HbF):
A type of hemoglobin predominant in the fetus, consisting of two alpha (α) and two gamma (γ) globin chains (α₂γ₂).
Structure and Function
- Higher Oxygen Affinity:
HbF has a greater affinity for oxygen compared to adult hemoglobin (HbA, α₂β₂), enabling efficient oxygen uptake from the maternal blood across the placenta.
Physiological Significance
- Placental Gas Exchange:
Facilitates the transfer of oxygen from maternal blood (low HbF affinity) to fetal blood (high HbF affinity), optimizing oxygen uptake by fetal tissues. - Efficient Oxygen Transfer:
The high affinity of HbF for oxygen ensures that the developing fetus receives adequate oxygen from the mother’s blood, even when maternal oxygen levels are lower.
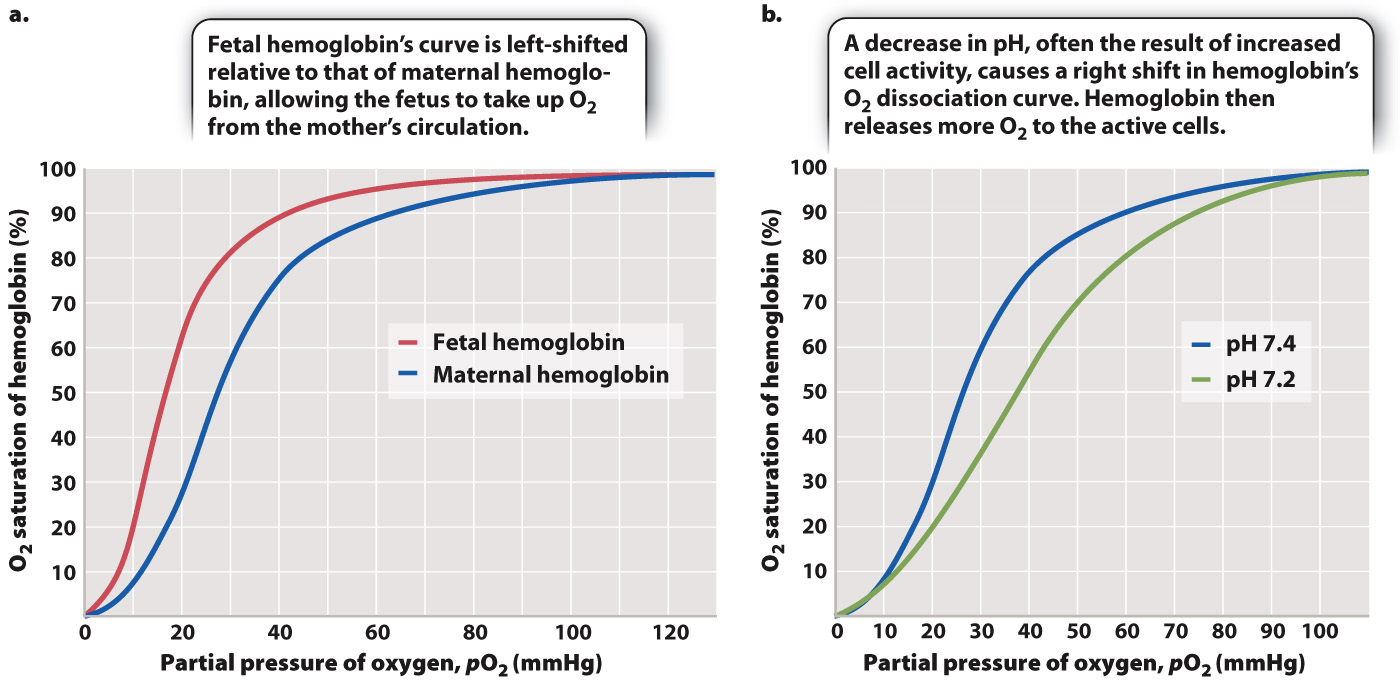
Carbon Dioxide Transport in Blood
- As Hydrogencarbonate Ions (HCO₃⁻):
- 85% of CO₂ is converted to HCO₃⁻ ions in red blood cells.
- Chloride Shift: HCO₃⁻ diffuses out of cells; Cl⁻ ions enter to maintain ionic balance.
- Dissolved CO₂ in Plasma:
- 5% of CO₂ remains as dissolved molecules in blood plasma.
- As Carbaminohaemoglobin:
- 10% of CO₂ binds directly to haemoglobin in red blood cells, forming carbaminohaemoglobin.
- Reverse Reaction in Lungs:
- Lower CO₂ concentration in lungs drives the reverse reaction, releasing CO₂ from blood to alveoli for exhalation.
Key Terms in CO₂ Transport
- Carbonic Anhydrase:
- An enzyme in red blood cells that catalyzes the reaction converting CO₂ and H₂O to carbonic acid (H₂CO₃).
- Chloride Shift:
- The exchange of Cl⁻ ions for HCO₃⁻ ions between plasma and red blood cells to maintain ionic balance.
- Carbaminohaemoglobin:
- A compound formed when CO₂ binds directly to haemoglobin.
Common Errors in Understanding Gas Transport
- Oxyhaemoglobin Release:
- Incorrect: Oxyhaemoglobin gradually releases O₂ as it passes from the lungs to a muscle.
- Correct: O₂ is primarily released in tissues where the partial pressure of O₂ is low.
- Function of Arteries:
- Incorrect: Arteries pump blood around the body.
- Correct: Arteries transport blood; the heart pumps it.
- Red Blood Cells Surface Area:
- Incorrect: Red blood cells have a large surface area for oxygen attachment.
- Correct: RBCs’ surface area aids in diffusion of O₂, not direct attachment.
- CO₂ Transport:
- Incorrect: Most CO₂ is transported in plasma solution.
- Correct: Most CO₂ is transported as HCO₃⁻ ions, not dissolved directly.
Practice Questions
Question 1
Describe the structure of haemoglobin and explain how it facilitates oxygen transport in the blood. (5 marks)
Mark Scheme:
- Composition:
- Haemoglobin is a protein composed of four polypeptide chains, each containing one haem group that can bind one O₂ molecule. (1 mark)
- Oxygen Binding Capacity:
- Each haemoglobin molecule can bind up to four O₂ molecules (Hb + 4O₂ → HbO₄), allowing efficient oxygen transport. (1 mark)
- Biconcave Red Blood Cells:
- Haemoglobin is contained within red blood cells (erythrocytes), which have a biconcave shape increasing surface area for gas exchange. (1 mark)
- Cooperative Binding:
- Binding of one O₂ molecule changes haemoglobin’s shape, making it easier for additional O₂ molecules to bind, enhancing overall oxygen uptake. (1 mark)
- Transport Function:
- Haemoglobin transports oxygen from the lungs to body tissues for aerobic respiration and assists in the transport of carbon dioxide from tissues to the lungs. (1 mark)
Question 2
Explain the significance of the haemoglobin dissociation curve being S-shaped. (5 marks)
Mark Scheme:
- Cooperative Binding:
- The S-shaped (sigmoidal) curve indicates cooperative binding of oxygen; binding of one O₂ molecule increases the affinity for subsequent O₂ molecules. (1 mark)
- High Affinity at High pO₂:
- At high oxygen partial pressures (e.g., in the lungs), haemoglobin is highly saturated with oxygen, facilitating effective oxygen uptake. (1 mark)
- Low Affinity at Low pO₂:
- At low oxygen partial pressures (e.g., in tissues), haemoglobin releases oxygen more readily, ensuring oxygen delivery where it is needed most. (1 mark)
- Efficient Oxygen Transport:
- The shape allows haemoglobin to pick up oxygen efficiently in the lungs and release it efficiently in the tissues, optimizing gas transport. (1 mark)
- Adaptability to Metabolic Needs:
- The curve’s shape allows haemoglobin to adapt to varying oxygen demands, responding to changes in pO₂ and pH levels in different environments. (1 mark)
Question 3
Define the Bohr shift and describe its role in oxygen delivery to tissues. (5 marks)
Mark Scheme:
- Definition of Bohr Shift:
- The Bohr shift refers to the rightward shift of the haemoglobin dissociation curve under conditions of high CO₂ levels and low pH. (1 mark)
- Influence of CO₂ and pH:
- High CO₂ levels in active tissues increase H⁺ ion concentration (lower pH), which binds to haemoglobin and induces a conformational change. (1 mark)
- Reduced Affinity for O₂:
- This conformational change reduces haemoglobin’s affinity for oxygen, making it easier to release O₂ in tissues where it is most needed. (1 mark)
- Enhanced Oxygen Delivery:
- Facilitates the release of oxygen in CO₂-rich, metabolically active tissues (e.g., muscles during exercise), ensuring adequate oxygen supply for aerobic respiration. (1 mark)
- Physiological Significance:
- The Bohr shift enhances oxygen unloading in areas with high metabolic activity, optimizing oxygen delivery based on the body’s dynamic needs. (1 mark)
Question 4
Explain how carbon dioxide is transported in the blood. Include the roles of bicarbonate ions, carbaminohaemoglobin, and dissolved CO₂. (6 marks)
Mark Scheme:
- As Bicarbonate Ions (HCO₃⁻):
- 85% of CO₂ is converted to HCO₃⁻ ions in red blood cells via the enzyme carbonic anhydrase. (1 mark)
- Chloride Shift: HCO₃⁻ ions diffuse out of red blood cells into plasma, while Cl⁻ ions enter red blood cells to maintain ionic balance. (1 mark)
- As Carbaminohaemoglobin:
- 10% of CO₂ binds directly to haemoglobin, forming carbaminohaemoglobin. (1 mark)
- Dissolved CO₂ in Plasma:
- 5% of CO₂ remains as dissolved molecules in blood plasma. (1 mark)
- Reverse Reaction in Lungs:
- In the lungs, the lower CO₂ concentration drives the reverse reaction, releasing CO₂ from HCO₃⁻ ions and carbaminohaemoglobin for exhalation. (1 mark)
- Role of Carbonic Anhydrase:
- Carbonic anhydrase catalyzes the conversion of CO₂ and H₂O to H₂CO₃, facilitating CO₂ transport. (1 mark)
- Chloride Shift Significance:
- Maintains ionic balance by exchanging Cl⁻ ions for HCO₃⁻ ions, ensuring blood remains electrically neutral during CO₂ transport. (1 mark)
Question 5
Describe the key features of the haemoglobin dissociation curve and how it relates to oxygen delivery in different parts of the body. (6 marks)
Mark Scheme:
- S-Shaped Curve:
- The haemoglobin dissociation curve is S-shaped (sigmoidal), indicating cooperative binding of oxygen. (1 mark)
- Low Oxygen Partial Pressure:
- At low pO₂ (e.g., in tissues), haemoglobin has low saturation, releasing oxygen where it is needed. (1 mark)
- High Oxygen Partial Pressure:
- At high pO₂ (e.g., in lungs), haemoglobin approaches full saturation (95-97%), effectively binding oxygen. (1 mark)
- Cooperative Binding:
- Binding of one O₂ molecule increases haemoglobin’s affinity for additional O₂ molecules, resulting in the steep rise in the curve. (1 mark)
- Bohr Shift Impact:
- Under high CO₂ conditions, the curve shifts right, reducing haemoglobin’s affinity for O₂ and promoting oxygen release in tissues. (1 mark)
- Physiological Relevance:
- The dissociation curve illustrates how haemoglobin efficiently binds oxygen in the lungs and releases oxygen in tissues, ensuring effective gas transport based on varying oxygen needs. (1 mark)
Question 6
Identify and explain the common errors students might make when understanding haemoglobin’s role in gas transport. (5 marks)
Mark Scheme:
- Oxyhaemoglobin Release:
- Incorrect: Oxyhaemoglobin gradually releases O₂ as it passes from the lungs to a muscle.
- Correct Explanation: O₂ is primarily released in tissues where the partial pressure of O₂ is low, not based on distance. (1 mark)
- Function of Arteries:
- Incorrect: Arteries pump blood around the body.
- Correct Explanation: Arteries transport blood away from the heart; the heart is the pump. (1 mark)
- Red Blood Cells Surface Area:
- Incorrect: Red blood cells have a large surface area for oxygen attachment.
- Correct Explanation: RBCs’ surface area aids in the diffusion of O₂, not direct attachment. (1 mark)
- CO₂ Transport:
- Incorrect: Most CO₂ is transported in plasma solution.
- Correct Explanation: Most CO₂ is transported as HCO₃⁻ ions, not dissolved directly in plasma. (1 mark)
- Haemoglobin Binding Capacity:
- Incorrect: Haemoglobin can bind unlimited O₂ molecules.
- Correct Explanation: Each haemoglobin molecule can bind up to four O₂ molecules. (1 mark)
Question 7
Explain how the structure of red blood cells enhances their function in gas exchange. (5 marks)
Mark Scheme:
- Biconcave Shape:
- Red blood cells have a biconcave shape, increasing their surface area relative to volume, facilitating efficient gas diffusion. (1 mark)
- Lack of Nucleus:
- They lack a nucleus, providing more space for haemoglobin molecules to bind oxygen. (1 mark)
- Flexible Membrane:
- The flexible cell membrane allows RBCs to pass through narrow capillaries easily, ensuring continuous gas exchange. (1 mark)
- High Haemoglobin Content:
- Rich in haemoglobin, RBCs can carry a large amount of oxygen bound to haemoglobin molecules. (1 mark)
- No Organelles:
- Absence of organelles reduces metabolic demands, allowing RBCs to focus on gas transport. (1 mark)
Question 8
Describe how carbon dioxide is transported in the blood and the role of the chloride shift in this process. (6 marks)
Mark Scheme:
- As Hydrogencarbonate Ions (HCO₃⁻):
- 85% of CO₂ is converted to HCO₃⁻ ions in red blood cells via carbonic anhydrase. (1 mark)
- Chloride Shift: HCO₃⁻ ions diffuse out of red blood cells into plasma, while Cl⁻ ions enter to maintain ionic balance. (1 mark)
- Dissolved CO₂ in Plasma:
- 5% of CO₂ remains as dissolved molecules in blood plasma. (1 mark)
- As Carbaminohaemoglobin:
- 10% of CO₂ binds directly to haemoglobin, forming carbaminohaemoglobin. (1 mark)
- Reverse Reaction in Lungs:
- In the lungs, the lower CO₂ concentration drives the reverse reaction, releasing CO₂ from HCO₃⁻ ions and carbaminohaemoglobin for exhalation. (1 mark)
- Role of Chloride Shift:
- Maintains ionic balance by exchanging Cl⁻ ions for HCO₃⁻ ions, ensuring blood remains electrically neutral during CO₂ transport. (1 mark)
- Overall Transport Mechanism:
- Ensures efficient removal of CO₂ from tissues and its transport to the lungs for exhalation. (1 mark)
Question 9
Draw and label a haemoglobin dissociation curve, explaining how changes in partial pressure of oxygen and carbon dioxide affect oxygen release. (6 marks)
Mark Scheme:
- Accurate Drawing of S-Shaped Curve:
- A correctly drawn S-shaped (sigmoidal) curve showing oxygen saturation on the Y-axis and oxygen partial pressure (pO₂) on the X-axis. (1 mark)
- High pO₂ (Lungs):
- At high pO₂, haemoglobin is fully saturated with O₂ (left side of the curve). (1 mark)
- Low pO₂ (Tissues):
- At low pO₂, haemoglobin releases O₂ (right side of the curve). (1 mark)
- Bohr Shift Illustration:
- Curve shifts right under high CO₂ conditions, indicating lower affinity for O₂ and enhanced release in tissues. (1 mark)
- Label Key Points:
- Indicate Oxygen Binding in lungs and Oxygen Release in tissues. (1 mark)
- Explanation of Effects:
- Explain that high pO₂ facilitates O₂ binding in the lungs, while high CO₂ and low pO₂ in tissues promote O₂ release through the Bohr Shift. (1 mark)
Question 10
Explain the role of carbonic anhydrase in the transport of carbon dioxide in the blood. (5 marks)
Mark Scheme:
- Enzymatic Function:
- Carbonic anhydrase is an enzyme located in red blood cells that catalyzes the reaction of CO₂ with H₂O to form carbonic acid (H₂CO₃). (1 mark)
- Reaction Facilitation:
- Accelerates the conversion of CO₂ + H₂O → H₂CO₃ → H⁺ + HCO₃⁻, facilitating the efficient transport of CO₂ as bicarbonate ions. (1 mark)
- Formation of Bicarbonate Ions:
- Enables the majority of CO₂ to be transported in the blood as HCO₃⁻ ions, which is more efficient than transport as dissolved CO₂. (1 mark)
- Chloride Shift Assistance:
- The production of HCO₃⁻ ions is linked to the chloride shift, maintaining ionic balance by exchanging Cl⁻ ions into red blood cells. (1 mark)
- Overall Significance:
- Ensures the rapid and efficient removal of CO₂ from tissues and its transport to the lungs for exhalation. (1 mark)
Teaching Resources