13.21 Chapter Summary
BioCast
1. Structure and Function of Chloroplasts
Chloroplast Structure:
- Outer Membrane: Smooth membrane that encloses the chloroplast.
- Inner Membrane: Folded to form structures called thylakoids.
- Thylakoids: Flattened sacs where the light-dependent reactions occur. They are stacked into grana (singular: granum).
- Stroma: The fluid-filled space surrounding the thylakoids; site of the light-independent reactions (Calvin cycle).
- Granum (plural: Grana): Stacks of thylakoids that increase the surface area for light absorption.
Function Relationship:
- Thylakoids and Grana: The extensive folding into grana allows for a greater surface area to house chlorophyll and other pigments, maximizing light absorption for the light-dependent reactions.
- Stroma: Contains enzymes, such as Rubisco, necessary for the Calvin cycle, facilitating the synthesis of glucose from carbon dioxide.
Visual Aids:
- Diagrams: Chloroplast structure, highlighting thylakoids, grana, and stroma.
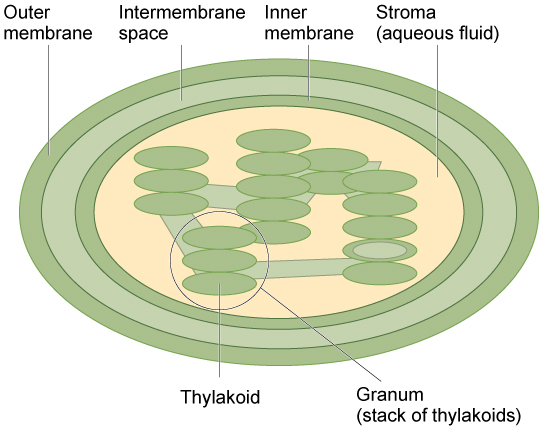
- Electron Micrographs: Organization of thylakoids within grana.
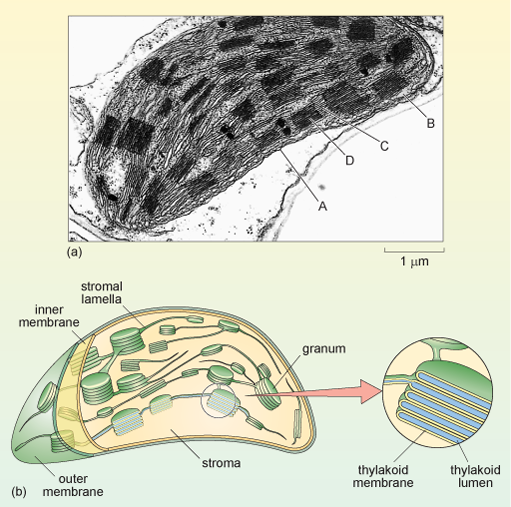
2. Energy Transfer from Light-Dependent to Light-Independent Reactions
Light-Dependent Reactions:
- Occur in the thylakoid membranes.
- Convert light energy into chemical energy, producing ATP and NADPH.
Light-Independent Reactions (Calvin Cycle):
- Occur in the stroma.
- Use ATP and NADPH to fix carbon dioxide into glucose and other organic molecules.
Energy Transfer Mechanism:
- ATP (Adenosine Triphosphate): Acts as an energy carrier, providing the necessary energy for the synthesis of glucose.
- NADPH (Reduced Nicotinamide Adenine Dinucleotide Phosphate): Provides reducing power (electrons) for the reduction of carbon dioxide during glucose synthesis.
Summary: Energy captured in the light-dependent reactions is stored in ATP and NADPH, which are then utilized in the Calvin cycle to produce complex organic molecules essential for plant growth and metabolism.
3. Sites of Light-Dependent and Light-Independent Stages within the Chloroplast
Thylakoids and Grana:
- Location of Light-Dependent Reactions: The thylakoid membranes within grana are the primary sites where light energy is converted into ATP and NADPH.
Stroma:
- Location of Light-Independent Reactions: The stroma houses the enzymes necessary for the Calvin cycle, where ATP and NADPH are used to synthesize glucose.
Visualization:
- Chloroplast Cross-Section: Thylakoid stacks (grana) and the surrounding stroma.
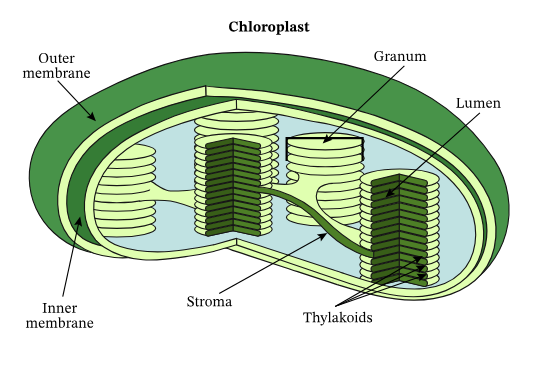
4. Role of Chloroplast Pigments in Light Absorption
Primary Pigments:
- Chlorophyll a: The main pigment involved in photosynthesis, absorbs primarily blue-violet and red light.
- Chlorophyll b: Accessory pigment that absorbs blue and red-orange light, complementing chlorophyll a.
- Carotene and Xanthophyll: Accessory pigments that absorb blue and green light, protecting chlorophyll from excessive light and aiding in light capture.
Function in Thylakoids:
- These pigments are embedded in the photosystems within the thylakoid membranes.
- They capture light energy and transfer it to the reaction centers (chlorophyll a) where it is converted into chemical energy.
Pigment Arrangement:
- Photosystem I (PSI): Contains chlorophyll a and b, absorbs light primarily in the red region.
- Photosystem II (PSII): Contains chlorophyll a and accessory pigments like carotene and xanthophyll, absorbs light primarily in the blue region.
5. Absorption and Action Spectra Interpretation
Absorption Spectrum:
- Graph showing the wavelengths of light absorbed by chloroplast pigments.
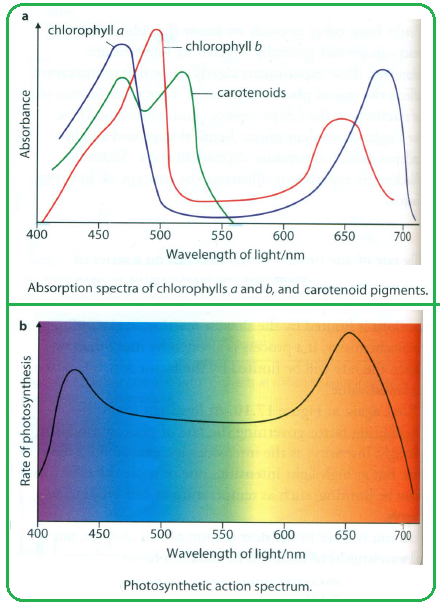
- Chlorophyll a: Peaks around 430 nm (blue) and 662 nm (red).
- Chlorophyll b: Peaks around 453 nm (blue) and 642 nm (red).
- Carotenoids: Peaks around 450 nm (blue) and less absorption in the green spectrum.
Interpretation:
- The overlap between absorption and action spectra confirms that the pigments absorbed light energy, which is then utilized in the photosynthetic process.
6. Chromatography for Separation and Identification of Chloroplast Pigments
Chromatography Technique:
- Paper Chromatography: Used to separate pigments based on their solubility and interaction with the solvent.
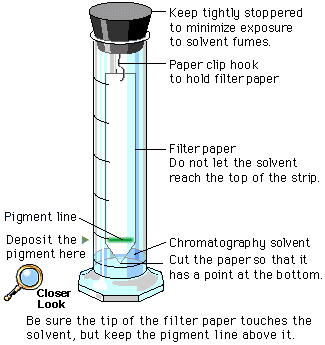
Procedure:
- Extract pigments from chloroplasts using a solvent (e.g., acetone).
- Spot the extract on chromatography paper.
- Place the paper in a solvent chamber, allowing the solvent to travel up the paper via capillary action.
- Pigments separate based on their affinity to the solvent and paper.
Rf Values (Retention Factors):
- Definition: Ratio of the distance traveled by the pigment to the distance traveled by the solvent front.
- Usage: Compare Rf values of unknown pigments with known standards to identify chlorophyll a, chlorophyll b, carotene, and xanthophyll.
Identification:
- Each pigment has a characteristic Rf value, allowing for accurate identification post-separation.
7. Cyclic and Non-Cyclic Photophosphorylation
Overview:
- Photophosphorylation: The process of converting ADP to ATP using the energy of sunlight during the light-dependent reactions.
- Two Pathways:
- Cyclic Photophosphorylation
- Non-Cyclic Photophosphorylation
8. Cyclic Photophosphorylation
Characteristics:
- Involves Only PSI: Electrons cycle back to PSI after passing through the electron transport chain.
- Photoactivation of Chlorophyll: Light energy excites electrons in chlorophyll a of PSI.
- ATP Synthesis: The energy released as electrons move through the transport chain is used to pump protons and generate ATP via ATP synthase.
Process Steps:
- Light energy excites electrons in PSI.
- Excited electrons move through the electron transport chain.
- Energy from electrons is used to pump protons into the thylakoid space, creating a proton gradient.
- Protons flow back into the stroma through ATP synthase, driving ATP production.
- Electrons return to PSI, completing the cycle.
Outcome:
- Production of ATP without the production of NADPH or oxygen.
9. Non-Cyclic Photophosphorylation
Characteristics:
- Involves PSI and PSII: Electrons flow from PSII to PSI without returning to PSII.
- Photoactivation of Chlorophyll: Light energy excites electrons in both PSII and PSI.
- Oxygen-Evolving Complex: Located in PSII, catalyzes the splitting of water molecules (photolysis) to replace lost electrons, releasing oxygen as a byproduct.
- ATP and NADPH Synthesis: Energy from electron transport is used to produce ATP and reduce NADP⁺ to NADPH.
Process Steps:
- Light energy excites electrons in PSII, which are transferred to the electron transport chain.
- Water is split by the oxygen-evolving complex to replace lost electrons, releasing O₂.
- Electrons travel through the transport chain to PSI.
- Light energy excites electrons in PSI, which are ultimately used to reduce NADP⁺ to NADPH.
- Proton gradient established by electron transport drives ATP synthesis via ATP synthase.
Outcome:
- Production of ATP, NADPH, and oxygen.
10. Photophosphorylation Mechanisms
Energy Release and Proton Gradient:
- Electron Transport Chain (ETC): As electrons move through the ETC, energy is released.
- Proton Pumping: This energy is utilized to pump protons (H⁺) across the thylakoid membrane into the thylakoid space, creating a high concentration of protons inside.
ATP Synthesis:
- Proton Flow: Protons flow back into the stroma through ATP synthase via facilitated diffusion.
- Chemiosmosis: The movement of protons drives the synthesis of ATP from ADP and inorganic phosphate (Pi).
Summary of Steps:
- Excited electrons release energy as they move through the ETC.
- Energy is used to pump protons across the thylakoid membrane, establishing a proton gradient.
- Protons flow back through ATP synthase, providing the energy required for ATP production.
Note:
- ATP Synthase Details: While not required, it’s important to understand that ATP synthase functions like a molecular turbine, harnessing proton flow to catalyze ATP formation.
11. The Calvin Cycle: Three Main Stages
- Overview: The Calvin cycle is a series of biochemical reactions that take place in the stroma of chloroplasts during the light-independent reactions of photosynthesis. It fixes carbon dioxide into organic molecules, ultimately producing glucose.
Stage 1: Carbon Fixation
- Enzyme Involved: Rubisco (Ribulose-1,5-bisphosphate carboxylase/oxygenase).
- Process:
- Rubisco catalyzes the fixation of CO₂ by attaching it to ribulose bisphosphate (RuBP), a five-carbon compound.
- The reaction yields an unstable six-carbon compound that immediately splits into two molecules of glycerate 3-phosphate (GP), each with three carbon atoms.
Stage 2: Reduction Phase
- Processes:
- GP Reduction: Glycerate 3-phosphate is phosphorylated by ATP and then reduced by NADPH to form triose phosphate (TP).
- NADPH Role: Provides the necessary electrons (reducing power) for the reduction of GP to TP.
- ATP Role: Provides the energy required for the phosphorylation steps.
Stage 3: Regeneration of RuBP
- Process:
- Some triose phosphate molecules are used to regenerate RuBP, enabling the cycle to continue.
- This regeneration requires additional ATP molecules.
- Enzymatic Steps: A series of reactions rearrange the carbon skeletons of TP to regenerate RuBP.
Overall Outcome:
- Glucose Production: While the Calvin cycle primarily produces TP, these molecules are used to synthesize glucose and other carbohydrates.
- Cycle Continuation: The regeneration of RuBP ensures the cycle can continue as long as there is sufficient ATP and NADPH.
12. Utilization of Calvin Cycle Intermediates
Intermediates:
- Glycerate 3-Phosphate (GP): Can be used to synthesize amino acids.
- Triose Phosphate (TP): Serves as a precursor for various biomolecules.
Biosynthetic Pathways:
- Amino Acids: Synthesized from GP, which provides carbon skeletons for amino acid biosynthesis.
- Carbohydrates: TP can be converted into glucose and other carbohydrates, essential for energy storage and structural functions.
- Lipids: TP serves as a building block for fatty acid synthesis, crucial for membrane formation and energy storage.
- Amino Acids and Lipids: Further metabolism of TP leads to the synthesis of diverse amino acids and lipids, supporting various cellular functions.
Integration into Cellular Metabolism:
- The Calvin cycle intermediates link photosynthesis to other metabolic pathways, ensuring that the plant can synthesize a wide array of necessary organic compounds.
Additional Key Concepts
Photosystems:
- Photosystem I (PSI): Absorbs light primarily in the red region, involved in the production of NADPH.
- Photosystem II (PSII): Absorbs light primarily in the blue region, involved in water splitting and oxygen release.
Photolysis of Water:
- Occurs in PSII during non-cyclic photophosphorylation.
- Splits water molecules into oxygen, protons, and electrons.
- Equation: 2 H₂O → 4 H⁺ + 4 e⁻ + O₂
Rubisco Efficiency:
- Rubisco can also catalyze a reaction with oxygen (photorespiration), which is less efficient than carbon fixation.
- Plants have mechanisms to minimize photorespiration, such as C₄ and CAM pathways, though these are beyond the scope of this syllabus.
Chloroplast Pigment Functions:
- Light Absorption: Pigments capture specific wavelengths of light, initiating the energy transfer processes.
- Energy Transfer: Excited electrons from pigments are transferred to reaction centers, starting the electron transport chain.
Energy Conversion:
- Light-Dependent Reactions: Convert light energy into chemical energy (ATP and NADPH).
- Light-Independent Reactions (Calvin Cycle): Convert chemical energy into stored organic molecules (glucose).
13. Limiting Factors of Photosynthesis
- Definition: Limiting factors are environmental conditions that restrict the rate at which photosynthesis occurs. When one or more of these factors are not at optimal levels, the efficiency of photosynthesis can be significantly reduced.
Key Limiting Factors:
- Light Intensity:
- Provides the energy required for photosynthesis.
- Absorption of light by chlorophyll initiates the light-dependent reactions.
- Carbon Dioxide Concentration:
- CO₂ is a raw material for the Calvin cycle (light-independent reactions) where it is fixed into glucose.
- Temperature:
- Affects the enzymes involved in photosynthesis.
- Optimal temperature ensures maximal enzyme activity, while deviations can reduce efficiency.
Other Potential Limiting Factors (for comprehensive understanding):
- Water Availability:
- Essential for photosynthesis and maintaining cell turgidity.
- Nutrient Availability:
- Elements like nitrogen, phosphorus, and potassium are vital for plant growth and enzyme function.
14. Effects of Limiting Factors on the Rate of Photosynthesis
a. Light Intensity:
- Low Light Intensity:
- Reduced energy for the light-dependent reactions.
- Decreased rate of ATP and NADPH production, limiting the Calvin cycle.
- High Light Intensity:
- Increases the rate of photosynthesis up to a saturation point.
- Beyond saturation, the rate plateaus as other factors become limiting.
b. Carbon Dioxide Concentration:
- Low CO₂ Concentration:
- Limits the Calvin cycle’s ability to fix carbon.
- Reduces glucose synthesis, slowing overall photosynthesis rate.
- High CO₂ Concentration:
- Enhances the rate of photosynthesis until the enzymes or other factors become limiting.
c. Temperature:
- Low Temperature:
- Slows down enzymatic reactions in both light-dependent and light-independent stages.
- Reduces overall photosynthetic rate.
- High Temperature:
- Increases reaction rates up to an optimal point.
- Excessive temperatures can denature enzymes, drastically reducing photosynthesis.
Graphs and Models:
- Michaelis-Menten Kinetics:
- Often used to describe the relationship between substrate concentration (S) (e.g., CO₂) and reaction rate.
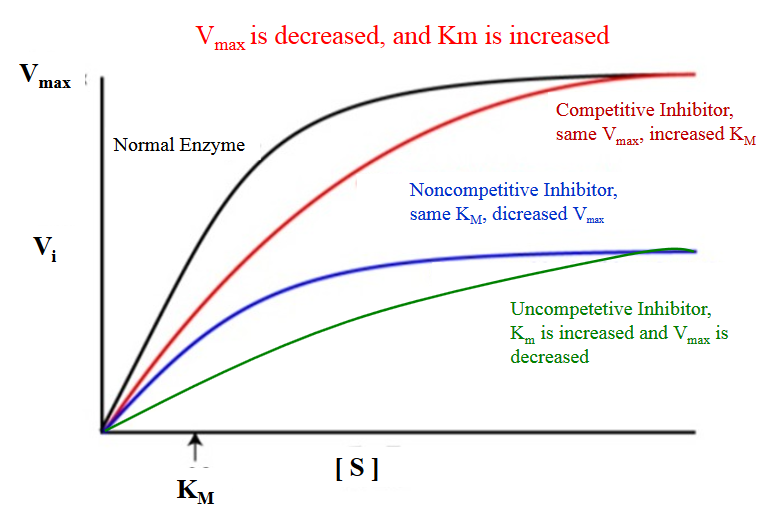
- Light Response Curves:
- The relationship between light intensity and photosynthesis rate, showing saturation.
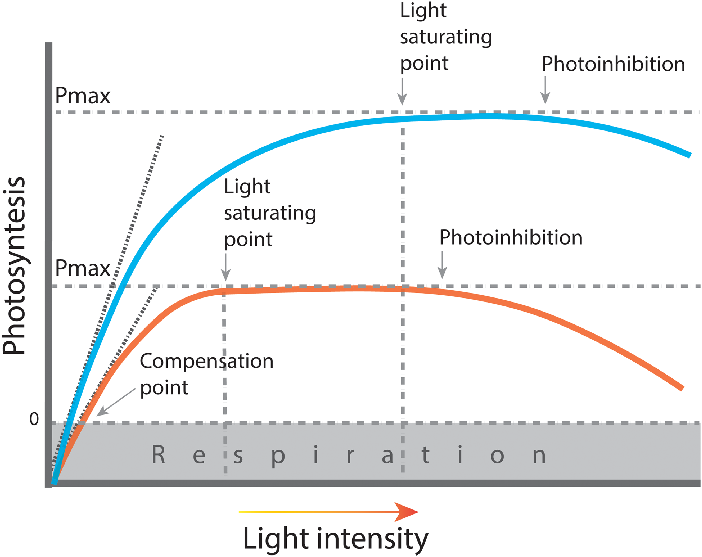
15. Investigations Using Redox Indicators and Chloroplast Suspensions
a. Redox Indicators:
- DCPIP (2,6-Dichlorophenol-indophenol):
- Blue in oxidized form; turns colorless when reduced.
- Used to measure the rate of photosynthesis by monitoring the reduction of DCPIP as electrons are transferred during the light-dependent reactions.
- Methylene Blue:
- Blue in oxidized form; becomes colorless upon reduction.
- Acts similarly to DCPIP in indicating photosynthetic activity.
b. Chloroplast Suspensions:
- Preparation:
- Extract chloroplasts from spinach leaves or other green plant tissues using homogenization and centrifugation.
- Procedure:
- Mix chloroplast suspension with DCPIP or methylene blue.
- Expose the mixture to varying light intensities or different wavelengths using filters.
- Observe and record the rate at which the indicator changes color, indicating the rate of photosynthesis.
c. Determining Effects of Light Intensity and Wavelength:
- Light Intensity:
- Vary the distance between the light source and the chloroplast suspension.
- Measure the rate of color change of the indicator at each distance.
- Light Wavelength:
- Use colored filters (e.g., red, blue, green) to isolate specific wavelengths.
- Assess which wavelengths maximize the rate of photosynthesis by observing the indicator’s color change rate.
d. Expected Results:
- Light Intensity:
- Increased light intensity leads to a faster rate of color change until saturation is reached.
- Light Wavelength:
- Blue and red wavelengths typically result in higher rates of photosynthesis due to chlorophyll absorption peaks.
e. Evaluation:
- Advantages:
- Direct visual indication of photosynthetic activity.
- Simple and cost-effective.
- Limitations:
- Indicators may have varying sensitivities.
- Chloroplasts may lose functionality over time.
16. Investigations Using Whole Plants (Including Aquatic Plants)
a. Use of Aquatic Plants (e.g., Elodea):
- Rationale:
- Aquatic plants are easy to observe as they release oxygen bubbles during photosynthesis, providing a visual measure of the rate.
b. Experimental Variables:
- Independent Variables:
- Light intensity
- CO₂ concentration
- Temperature
- Dependent Variable:
- Rate of photosynthesis (measured by oxygen bubble production)
- Controlled Variables:
- Type and size of plant
- Volume of water
- Duration of the experiment
c. Experimental Procedures:
- Effect of Light Intensity:
- Setup:
- Place identical Elodea plants in water with a consistent CO₂ source (e.g., bicarbonate solution).
- Position under varying light intensities using lamps at different distances.
- Measurement:
- Count the number of oxygen bubbles produced per minute.
- Expected Outcome:
- More bubbles (higher photosynthesis rate) with increased light intensity up to a saturation point.
- Setup:
- Effect of CO₂ Concentration:
- Setup:
- Place Elodea in water with varying concentrations of bicarbonate (source of CO₂).
- Maintain constant light intensity and temperature.
- Measurement:
- Count oxygen bubbles produced per minute.
- Expected Outcome:
- Increased CO₂ leads to more bubbles until CO₂ becomes non-limiting.
- Setup:
- Effect of Temperature:
- Setup:
- Incubate Elodea in water at different temperatures (using water baths).
- Keep light intensity and CO₂ concentration constant.
- Measurement:
- Count oxygen bubbles produced per minute.
- Expected Outcome:
- Higher temperatures increase photosynthesis rate up to an optimal point; beyond that, the rate declines.
- Setup:
d. Data Representation:
- Graphs:
- Plot photosynthesis rate (bubbles/min) against each independent variable.
- Analysis:
- Identify optimal conditions for maximum photosynthesis.
- Discuss deviations and potential experimental errors.
e. Evaluation:
- Advantages:
- Simple and direct measurement.
- Real-time observation of photosynthetic activity.
- Limitations:
- Oxygen bubble counting may be influenced by factors like plant health.
- Environmental fluctuations (e.g., room temperature changes) can affect results.
Additional Considerations for Exam Preparation
a. Understanding the Underlying Processes:
- Light-Dependent Reactions:
- Occur in the thylakoid membranes.
- Produce ATP and NADPH.
- Calvin Cycle (Light-Independent Reactions):
- Occur in the stroma.
- Fix CO₂ into glucose using ATP and NADPH.
b. Chlorophyll and Light Absorption:
- Chlorophyll a vs. Chlorophyll b:
- Absorption spectra differ slightly, allowing plants to utilize a broader range of light wavelengths.
- Accessory Pigments:
- Carotenoids help in capturing light energy and protecting chlorophyll from photo-damage.
c. Enzyme Activity and Temperature:
- Optimal Temperature Range:
- Typically between 15°C and 25°C for many plants.
- Enzyme Denaturation:
- At high temperatures, enzymes lose their functional shape, reducing photosynthetic efficiency.
d. Real-World Applications:
- Agriculture:
- Maximizing photosynthesis to increase crop yields by optimizing light, CO₂, and temperature conditions.
- Climate Change:
- Understanding how rising temperatures and changing CO₂ levels affect plant growth and ecosystem balance.