16.16 Chapter Summary
BioCast:
1. Haploid (n) and Diploid (2n)
- Haploid (n):
- Definition: A cell containing one complete set of chromosomes.
- Example: Gametes (sperm and egg cells).
- Significance: Ensures chromosome number is maintained across generations.
- Diploid (2n):
- Definition: A cell containing two complete sets of chromosomes, one from each parent.
- Example: Somatic (body) cells.
- Significance: Allows for genetic diversity and proper chromosome pairing during meiosis.
2. Homologous Pairs of Chromosomes
- Definition: Pairs of chromosomes that are similar in shape, size, and genetic content. One chromosome of each pair is inherited from each parent.
- Characteristics:
- Contain the same genes at the same loci, but may have different alleles.
- Pair up during meiosis I, specifically in prophase I.
- Example: Humans have 23 pairs of homologous chromosomes (46 in total).
3. Need for Reduction Division during Meiosis in Gamete Production
- Reduction Division (Meiosis I):
- Purpose: Reduces the chromosome number by half from diploid (2n) to haploid (n).
- Reason: Ensures that upon fertilization, the resulting zygote has the correct diploid number of chromosomes.
- Consequences of No Reduction:
- Chromosome number would double each generation, leading to genetic imbalances.
4. Behaviour of Chromosomes in Plant and Animal Cells during Meiosis
- Key Structures Involved:
- Nuclear Envelope: Breaks down during prophase I and prophase II; re-forms during telophase I and telophase II.
- Spindle Fibers: Formed from centrosomes (animal cells) or spindle poles (plant cells) to segregate chromosomes.
- Cell Surface Membrane: Undergoes cytokinesis to divide the cell into two daughter cells.
- Main Stages of Meiosis:
- Meiosis I:
- Prophase I: Homologous chromosomes pair up (synapsis) and crossing over occurs.
- Metaphase I: Homologous pairs align at the metaphase plate.
- Anaphase I: Homologous chromosomes are pulled to opposite poles.
- Telophase I: Nuclear envelopes may reform; cytokinesis divides the cell into two haploid cells.
- Meiosis II:
- Prophase II: Spindle fibers form in each haploid cell.
- Metaphase II: Chromosomes align individually at the metaphase plate.
- Anaphase II: Sister chromatids are separated to opposite poles.
- Telophase II: Nuclear envelopes reform; cytokinesis results in four genetically distinct haploid gametes.
- Meiosis I:
5. Interpreting Photomicrographs and Diagrams of Cells in Different Stages of Meiosis
- Identifying Stages:
- Prophase I: Chromosomes appear thickened and paired; crossing over visible as chiasmata.
- Metaphase I: Homologous pairs align side by side at the metaphase plate.
- Anaphase I: Homologous chromosomes move to opposite poles.
- Telophase I: Two haploid cells may show reformed nuclear envelopes.
- Prophase II: Similar to prophase but occurs in haploid cells.
- Metaphase II: Chromosomes align individually at the metaphase plate.
- Anaphase II: Sister chromatids separate.
- Telophase II: Four distinct haploid cells are formed.
- Tips for Interpretation:
- Look for Chromosome Pairing: Indicates prophase I.
- Observe Chromosome Alignment: Metaphase stages differ between I and II based on pairing.
- Note Separation Patterns: Homologous chromosomes separate in anaphase I; sister chromatids in anaphase II.
6. Crossing Over and Random Orientation (Independent Assortment)
- Crossing Over:
- Definition: Exchange of genetic material between non-sister chromatids of homologous chromosomes during prophase I.
- Effect: Creates new allele combinations, increasing genetic diversity.
- Result: Each gamete has a unique set of genes different from both parents and siblings.
- Independent Assortment:
- Definition: Random orientation of homologous chromosome pairs during metaphase I.
- Effect: Determines the combination of maternal and paternal chromosomes that go into each gamete.
- Result: Leads to numerous possible genetic combinations, enhancing variation.
- Genetic Outcome:
- Genetic Variation: Both crossing over and independent assortment contribute to genetically unique gametes.
7. Random Fusion of Gametes at Fertilisation Produces Genetically Different Individuals
- Fertilisation Process:
- Random Fusion: Any sperm can fuse with any egg, each carrying a unique combination of genetic information.
- Genetic Implications:
- Unique Zygote: The combination of two unique gametes results in a zygote with a distinct genetic makeup.
- Genetic Diversity: Ensures each individual is genetically different from their siblings (except identical twins) and parents.
Key Terms to Remember
- Haploid (n): Single set of chromosomes.
- Diploid (2n): Double set of chromosomes.
- Homologous Chromosomes: Pair of chromosomes with the same genes but possibly different alleles.
- Meiosis: The process of cell division that reduces chromosome number by half, resulting in gametes.
- Crossing Over: Exchange of genetic material between homologous chromosomes.
- Independent Assortment: Random distribution of maternal and paternal chromosomes into gametes.
- Gametes: Reproductive cells (sperm and egg) that are haploid.
- Zygote: The diploid cell formed by the fusion of gametes during fertilisation.
The Roles of Genes in Determining the Phenotype
8. Key Definitions
- Gene: A unit of heredity located on a chromosome that codes for a specific trait.
- Locus: The specific physical location of a gene on a chromosome.
- Allele: Different versions of a gene (e.g., A and a).
- Dominant Allele: Expressed in the phenotype even if only one copy is present (e.g., A).
- Recessive Allele: Expressed in the phenotype only when two copies are present (e.g., a).
- Codominant Alleles: Both alleles are fully expressed in the phenotype (e.g., AB blood type).
- Phenotype: The observable characteristics of an organism (e.g., flower color).
- Genotype: The genetic makeup of an organism (e.g., AA, Aa, aa).
- Homozygous: Having two identical alleles for a trait (e.g., AA or aa).
- Heterozygous: Having two different alleles for a trait (e.g., Aa).
- Linkage: When genes are located close to each other on the same chromosome and tend to be inherited together.
- Test Cross: A cross between an individual with an unknown genotype and a homozygous recessive individual to determine the unknown genotype.
- F₁ Generation: The first generation of offspring from a cross.
- F₂ Generation: The second generation of offspring, resulting from crossing individuals from the F₁ generation.
9. Genetic Diagrams and Punnett Squares
Monohybrid Cross
- Definition: A cross between two organisms focusing on a single trait.
- Punnett Square:
- Example: Crossing Aa × Aa for flower color.
- Results:
- Genotypes: AA, Aa, Aa, aa
- Phenotypes: 3 dominant, 1 recessive
Dihybrid Cross
- Definition: A cross between two organisms focusing on two traits.
- Punnett Square:
- Example: Crossing AaBb × AaBb for seed shape and color.
- Results:
- Genotypes: 9 A-B-, 3 A-bb, 3 aaB-, 1 aabb
- Phenotypes: 9 dominant for both traits, 3 dominant for one and recessive for the other, etc.
Codominance
- Definition: Both alleles in a gene pair are fully expressed.
- Example: AB blood type (A and B alleles both expressed).
Multiple Alleles
- Definition: More than two alleles exist for a gene.
- Example: ABO blood group system (A, B, O alleles).
Sex Linkage
- Definition: Genes located on sex chromosomes (X or Y).
- Example: Hemophilia is linked to the X chromosome.
Autosomal Linkage and Epistasis
- Autosomal Linkage: Genes located on non-sex chromosomes; may be inherited together.
- Epistasis: Interaction between genes where one gene masks or modifies the expression of another.
- Example: Coat color in Labrador Retrievers (B for black, b for brown; E allows color expression, e results in yellow regardless of B/b).
10. Test Crosses
- Purpose: To determine the genotype of an individual exhibiting the dominant phenotype.
- Procedure:
- Cross the individual with a homozygous recessive individual.
- Analyze the offspring’s phenotypes to infer the unknown genotype.
- Example:
- If unknown genotype is AA × aa → All offspring are Aa (phenotypically dominant).
- If unknown genotype is Aa × aa → Approximately 50% dominant, 50% recessive.
11. Chi-Squared Test
- Purpose: To determine if observed genetic data significantly deviates from expected ratios.
- Formula:
- O: Observed frequency
- E: Expected frequency
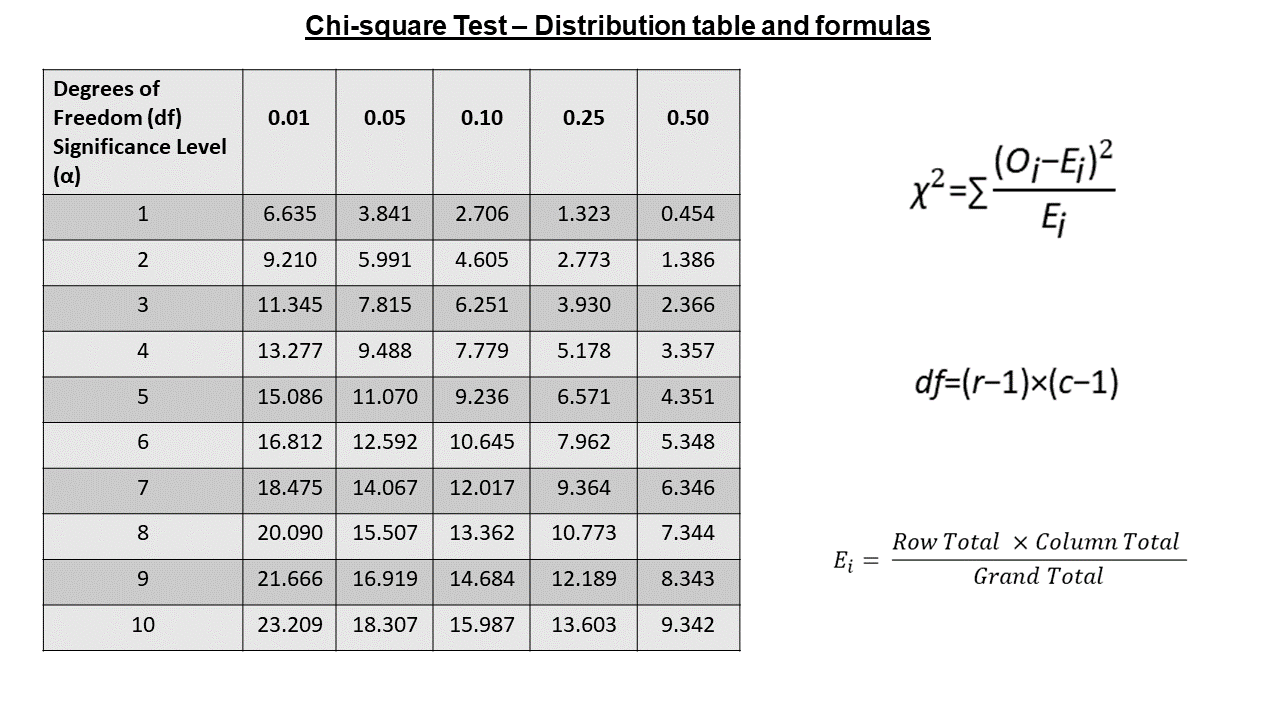
- Steps:
- Calculate expected frequencies based on genetic ratios.
- Use the formula to compute χ².
- Compare χ² to the critical value from χ² distribution tables to determine significance.
12. Gene-Protein-Phenotype Relationships
TYR Gene, Tyrosinase, and Albinism
- TYR Gene: Codes for the enzyme tyrosinase.
- Tyrosinase: Essential for melanin production.
- Albinism: Result of mutations in TYR leading to non-functional tyrosinase, resulting in lack of pigment.
HBB Gene, Haemoglobin, and Sickle Cell Anaemia
- HBB Gene: Codes for the β-globin subunit of haemoglobin.
- Haemoglobin: Protein in red blood cells carrying oxygen.
- Sickle Cell Anaemia: Mutation in HBB causes abnormal haemoglobin, leading to distorted (sickle-shaped) red blood cells.
F8 Gene, Factor VIII, and Haemophilia
- F8 Gene: Codes for clotting factor VIII.
- Factor VIII: Essential for blood clotting.
- Haemophilia: Mutation in F8 leads to deficient or dysfunctional factor VIII, causing prolonged bleeding.
HTT Gene, Huntingtin, and Huntington’s Disease
- HTT Gene: Codes for the huntingtin protein.
- Huntingtin: Involved in neuronal function.
- Huntington’s Disease: Mutation leads to faulty huntingtin protein, causing neurodegeneration and movement disorders.
13. Gibberellin and Stem Elongation
- Gibberellin: A plant hormone that promotes stem elongation.
- Alleles:
- Dominant Allele (Le):
- Codes for a functional enzyme in the gibberellin synthesis pathway.
- Results in normal stem elongation.
- Recessive Allele (le):
- Codes for a non-functional enzyme.
- Results in reduced or no stem elongation (dwarfism).
- Dominant Allele (Le):
- Genotypic Effects:
- LeLe or Lele: Tall stems (phenotypically dominant).
- lele: Dwarf stems (phenotypically recessive).
Example Problems
Monohybrid Cross:
- Question: Cross Aa × Aa for flower color (A = purple, a = white). What are the expected phenotypic ratios in F₂?
- Answer: 3 purple : 1 white.
- Dihybrid Cross with Codominance:
- Question: If A and B are codominant alleles, what is the phenotype of AaBb individuals?
- Answer: Both traits are expressed equally (e.g., red and white flowers resulting in pink flowers).
- Question: If A and B are codominant alleles, what is the phenotype of AaBb individuals?
Test Cross:
- Question: An individual with purple flowers (genotype unknown) is crossed with a white-flowered homozygous recessive. Offspring show a 1:1 ratio of purple to white. What is the unknown genotype?
- Answer: Aa (heterozygous).
- Chi-Squared Test:
- Question: Observed F₂ ratios: 90 dominant, 30 recessive. Expected ratio: 3:1. Is the difference significant?
- Calculation:
- Total: 120
- Expected: 90 dominant, 30 recessive.
- χ² = [(90-90)²/90] + [(30-30)²/30] = 0 + 0 = 0 → Not significant.
14. Structural Genes vs. Regulatory Genes
Structural Genes
- Function: Encode proteins that perform cellular functions (e.g., enzymes, structural proteins).
- Example: Genes that code for hemoglobin or insulin.
Regulatory Genes
- Function: Control the expression of structural genes.
- Mechanism: Produce proteins (regulators) that increase or decrease the transcription of specific structural genes.
- Example: LacI gene in the lac operon that codes for the repressor protein.
Repressible Enzymes vs. Inducible Enzymes
Repressible Enzymes
- Function: Typically involved in anabolic (biosynthetic) pathways.
- Regulation: Enzyme production is inhibited (repressed) when the end product is abundant.
- Example: Tryptophan synthesis in bacteria; high tryptophan levels repress the enzymes needed for its synthesis.
Inducible Enzymes
- Function: Usually involved in catabolic (breakdown) pathways.
- Regulation: Enzyme production is activated (induced) in the presence of a substrate.
- Example: Lactose metabolism in bacteria; presence of lactose induces enzymes for its breakdown.
15. Genetic Control of Protein Production in Prokaryotes: The Lac Operon
Components of the Lac Operon
- Structural Genes:
- lacZ: Codes for β-galactosidase.
- lacY: Codes for lactose permease.
- lacA: Codes for thiogalactoside transacetylase.
- Regulatory Elements:
- Promoter: Binding site for RNA polymerase.
- Operator: Binding site for the repressor protein.
- Regulator Gene (lacI): Produces the repressor protein.
Mechanism
- Absence of Lactose:
- Repressor protein binds to the operator.
- RNA polymerase cannot bind to the promoter.
- Structural genes are not transcribed; enzymes are not produced.
- Presence of Lactose:
- Lactose binds to the repressor, causing a conformational change.
- Repressor releases from the operator.
- RNA polymerase binds to the promoter and transcribes structural genes.
- Enzymes for lactose metabolism are produced.
16. Transcription Factors in Eukaryotes
Definition
- Transcription Factors: Proteins that bind to specific DNA sequences to regulate gene expression.
Functions
- Activation: Increase the rate of transcription by facilitating the binding of RNA polymerase to the promoter.
- Repression: Decrease the rate of transcription by blocking RNA polymerase access or by altering chromatin structure.
Mechanism
- Binding: Transcription factors bind to enhancer or silencer regions of DNA.
- Interaction: They interact with other proteins and the transcription machinery to modulate transcription rates.
- Outcome: Regulation of gene expression ensures appropriate protein levels for cellular function.
17. Gibberellin Activation of Genes in Eukaryotes
Role of Gibberellin
- Gibberellin: A plant hormone that promotes growth and influences various developmental processes.
Mechanism of Action
- Activation:
- Gibberellin binds to its receptor in the cell.
- Breakdown of DELLA Proteins:
- DELLA proteins are repressors that inhibit transcription factors involved in growth.
- Gibberellin signaling leads to the degradation of DELLA proteins.
- Promotion of Transcription:
- With DELLA repressors degraded, transcription factors are free to activate gene expression.
- Genes involved in growth and development are transcribed, leading to protein synthesis that promotes these processes.
Summary
- Without Gibberellin: DELLA proteins inhibit growth-promoting genes.
- With Gibberellin: DELLA proteins are degraded, allowing transcription factors to activate growth-promoting genes.
Key Points to Remember
- Structural vs. Regulatory Genes: Structural genes make proteins; regulatory genes control when and how much structural genes are expressed.
- Repressible vs. Inducible Enzymes: Repressible enzymes are shut off when their product is plentiful; inducible enzymes are turned on in the presence of a substrate.
- Lac Operon: A model for understanding gene regulation in prokaryotes; controlled by the presence or absence of lactose.
- Transcription Factors: Essential for regulating gene expression in eukaryotes by modulating transcription rates.
- Gibberellin and DELLA Proteins: Illustrates hormone-mediated gene activation by removing repression on transcription factors.